Cleanup
The cleanup of environmental pollution involves a variety of techniques, ranging from simple biological processes to advanced engineering technologies. Cleanup activities may address a wide range of contaminants, from common industrial chemicals such as petroleum products and solvents , agricultural chemicals and metals, to radionuclides . Cleanup technologies may be specific to the contaminant (or contaminant class) and to the site. This entry addresses the cleanup of contaminated soil and water. Air pollution is addressed generally at the point of release by control technologies, because the opportunities to capture and recover airborne contaminants are limited once they are released into the atmosphere.
Cleanup costs can vary dramatically depending on the contaminants, the media affected, and the size of the contaminated area. Much of the
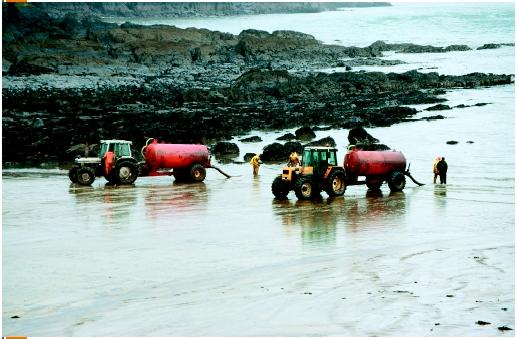
Many industry-specific cleanup programs (e.g., Florida's dry cleaner program) are funded by taxes or fees levied on that industry. Several Western European countries have environmental programs that are at least as aggressive as those in the United States. Countries with emerging economies are working hard to address environmental contamination with limited resources. Many cases of environmental contamination in former Warsaw Pact , for example, are associated with former Soviet military bases. In Poland, cleanup of several of these bases is under way. In Kluczewo, northwestern Poland, a former military base is reportedly the biggest and most contaminated such site in Central Europe. A skimming technique was used to remove liquid petroleum fuel from the subsurface followed by bioremediation of the remaining contaminated soil. The Polish government paid for the work with support from local sources.
Government involvement in environmental remediation includes consideration of the safety of the cleanup workers. Professionals involved in the cleanup of contaminated sites may have long-term exposure to a variety of hazardous materials and, as such, must be protected against adverse health
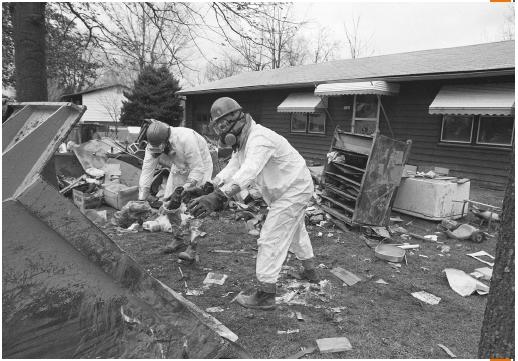
In most cases, it is financially or physically impractical to completely remove all traces of contamination. In such cases, it is necessary to set an acceptable level of residual contamination. Evaluating experimental toxicity data and then extrapolating to potential exposure scenarios forms the basis for such decisions. The result of these evaluations is an estimate of risk for given adverse outcome (e.g., cancer or death). Risk-based target levels typically determine when cleanup is complete. As a result, evolution of cleanup technologies has yielded four general categories of remediation approaches: (1) physical removal (with or without treatment); (2) in situ conversion by physical or chemical means to less toxic or less mobile forms; (3) containment ; and (4) passive cleanup, or natural attenuation . Combinations of technologies may be used at some sites.
Physical Removal
The physical removal of contaminated soil and groundwater has been, and continues to be, a common cleanup practice. However, physical removal does not eliminate the contamination, but rather transfers it to another location. In ideal cases, the other location will be a facility that is specially designed to contain the contamination for a sufficient period of time. In this way, proper removal reduces risk by reducing or removing the potential for exposure to the contamination. Removal options vary dramatically for soil and groundwater, as described below.
Soils. Excavation of contaminated soils works well for limited areas of contamination that are close to the ground surface. Under ideal conditions, the disposal location is a designed, regulated, and controlled disposal facility (e.g., a landfill or incineration facility). Alternatively, contaminated soil may be excavated and consolidated in a prepared facility on-site. Prepared disposal facilities range from simple excavations with impermeable covers (caps) to sophisticated containment structures such as those used in modern landfills. Landfills typically consist of multiple layers of impermeable materials—often combinations of synthetic (plastic) liners and compacted layers of dense clays; piping to collect and transport liquids generated within the landfill (leachate); and systems of sensors within and surrounding the landfill to detect leaks. When contaminated soil is excavated , transported, and disposed of properly, physical removal can be an effective and economical cleanup option.
Treatment of excavated soil, to either destroy the contaminant or to reduce its toxicity or mobility often is associated with physical removal. Treatment following removal will differ with the chemical of concern. Many organics (e.g., solvents, pesticides, oils) may be incinerated or landfilled effectively. Some metals require conversion to compounds that will not react with other substances before being transferred to a landfill. Treatment options also can be troublesome as landfill space decreases and public opposition to incineration increases in some areas. However, effective air pollution controls are available to manage incinerator emissions, and engineering for landfill construction now includes sophisticated liners, leachate controls, and management practices to prevent groundwater contamination or other forms of cross-pollution.
Beyond excavation, more selective removal technologies have been developed for contaminants in soil, including soil washing, which uses processing equipment and chemical solvents to "wash" contaminants from soil. In practice, soil washing often is complicated and expensive. Phytoextraction—the use of plants to remove soil contaminants—has achieved favor in some applications. Selected plant species may remove and concentrate inorganic contaminants such as heavy metals and radionuclides in the above- or below-ground tissues. If phytoextraction is successful, the resulting plant tissue will have high levels of the soil contaminant and be classified as hazardous waste, requiring appropriate treatment or disposal options (see previous section). To date, phytoextraction has been used only at relatively small sites.
One of the best-documented cases of heavy metal phytoremediation in the United States was conducted at a former battery manufacturing site in Trenton, New Jersey. The land surrounding this urban facility that was in operation from the 1930s until 1980 was highly contaminated with lead. For two vegetation seasons Indian mustard plants were used to reduce the concentration of lead in the soil to below regulatory limits. This cleanup effort illustrates the potential for innovative, biological remediation technologies.
Sediments are the inorganic (e.g., clay, silt, sand) and organic (plant and animal) materials that settle to the bottom of water bodies. Aquatic sediments often become contaminated by a wide variety of man-made chemicals including agricultural chemicals such as pesticides that are washed into water bodies, industrial chemicals that are released into water bodies or that leak from containment structures as well as the many products that are transported by water. Contamination in aquatic sediments may affect the organisms that live within the sediments, or may bioaccumulate through the food chain as larger species feed on organisms that have absorbed the contamination. Remediating such contamination requires choosing between the risks associated with leaving the contamination in place and the risks associated with excavating the sediments (and resuspending them in the water), transporting and disposing of them.
Groundwater. Liquid or solid chemicals, when disposed of by burial or direct release onto the ground surface, can migrate down into the soil structure and come in contact with groundwater. Final disposition of these chemicals depends on their volatility and water solubility. Aqueous phase chemicals, chemicals that are soluble in water, dissolve in and move with groundwater. Nonaqueous phase chemicals (NAPLs) do not dissolve in water and may be either lighter than water (light nonaqueous phase liquids or LNAPLs) or heavier than water (dense nonaqueous phase liquids or DNAPLs). The distinction between DNAPLs and LNAPLs has a significant impact on the detection and remediation of organic contamination.
LNAPLs such as petroleum products (e.g., gasoline, diesel, oils) are common contaminants in urban, industrial, and agricultural areas. DNAPLs such as chlorinated solvents—trichloroethylene (TCE) and perchloroethylene (PCE)—are found also in urban and industrial areas, most commonly in association with the dry cleaning industry, where previous management practices often resulted in the spilling or dumping of these chemicals. These NAPLs pool above (LNAPL) or below (DNAPL) groundwater bodies, dissolving slowly into, and potentially contaminating, enormous volumes of water. In states that rely heavily on groundwater for drinking water, billions of dollars have been spent in the last two decades to replace leaking underground gasoline storage tanks (LUSTs) and to clean up historical contamination.
When contamination is detected in groundwater, one common cleanup approach is to drill wells, then pump out and purify the contaminated water using a variety of methods, including air stripping , where compounds are volatized from the water into the air. This technique does not rid the environment of the pollutants, however, as the contaminants are merely transferred from the water to the air. Less volatile compounds, or those at low concentrations, may be removed by filtration through a solid sorbent , such as activated carbon. This "pump and treat" approach addresses only the dissolved, aqueous phase of contamination, while leaving the concentrated, nonaqueous "pool" as a continuing source of groundwater contamination. As a result, "pump and treat" may be a prolonged process. The detection and elimination of NAPL source zones of contamination are more desirable where feasible.
In order to remove sources of groundwater contamination, technologies are needed to accurately detect and measure the amounts of these chemicals. Well drilling is commonly used to investigate or remediate contaminated sites, though it is relatively slow and expensive, and it brings up contaminated soil that must be disposed of properly. Direct push technologies use large vehicles equipped with hydraulic rams or percussion equipment to push metal tubes into the ground. Special sensors on the advancing tip of these tubes provide information on the nature of the sediments being penetrated. Recent advances in this technology allow special chemical sensors to be deployed on the end of the tube providing information on the presence and concentration of chemicals in the ground. The hollow tube also can be used to collect soil and groundwater samples. When sampling is complete, the rods typically are removed from the ground and the hole is sealed. While depth and geology limit "direct push," it is generally faster than well drilling, and it does not contaminate the soil.
Once source zones have been identified, technologies may be deployed to remove contamination. One of the most popular approaches to removing NAPLs is thermal treatment. Heating contaminated soil and groundwater to the boiling point of the contaminant will convert liquids to gases, which move through the soil. Wells are used to extract the resulting gases that can then be absorbed by activated carbon, or heated to temperatures high enough to break them down into harmless elements. Typically, soils are heated in one of two ways: electrical resistance or steam injection. Electrical resistance heating uses electrodes placed in the ground between which electrical currents are passed. The soil's resistance to the movement of the electrical current produces heat. Steam heating pumps high-pressure steam into the ground through injection wells.
Conversion
Conversion uses chemical reactions to change contaminants into less toxic or less mobile forms. These chemical reactions may be produced by the introduction of reactive chemicals to the contaminated area, or by the action of living organisms such as bacteria.
The use of biological systems to clean up contamination is known as bioremediation . Bioremediation includes all cleanup technologies that take advantage of biological processes to remove contaminants from soil and groundwater; the most common technique is microbial metabolism. For decades, scientists have known that microbes can degrade certain organic contaminants, and in cases of historical contamination, microbial communities often adapt to take advantage of the energy released when these chemicals are degraded (i.e., metabolized). By studying the existing conditions, substances that microbes need to break down chemicals, such as nutrients or oxygen, may be added to enhance biodegradation. Microbial biodegradation is capable of degrading most organic contaminants.
For example, under ideal conditions, microbes can degrade the organic constituents of petroleum hydrocarbons such as gasoline or diesel fuel, to carbon dioxide and water. This is the concept behind a technology being used by the U.S. Department of Energy to remove petroleum contamination from soils that also contain low levels of radioactive materials. The combination of hazardous materials (petroleum) and radiation places this soil in the regulatory category of mixed waste, for which disposal is extremely difficult. By using biodegradation to remove the petroleum component, the remaining soil can be classified as low-level radioactive waste, which has an accepted disposal mechanism.
Soils. Heavy metals are a common target for conversion approaches. Removal may not be practical when such metals contaminate large areas of surface soil. In these cases, chemical approaches often are sought to convert the metals to a less toxic and less mobile form. Such conversions often involve the use of reactive agents such as sulfur to create immobile sulfide salts of metals (e.g., mercury). Reducing the mobility of soil contaminants often refers to reducing the water solubility of the compounds. Reducing water solubility lowers the potential for contaminants to become dissolved in and move with water in the subsurface.
Groundwater. DNAPLs such as chlorinated solvents may be treated with chemicals (e.g., potassium permanganate) that degrade the solvents into relatively harmless chemicals. When combined with chlorinated solvents, potassium permanganate removes chloride ions , which results in the degradation of these chemicals to carbon dioxide (CO 2 ) and water. This technology holds promise as a tool for remediating these challenging contaminants.
Containment
Situations exist in which technologies are not available or practical to remove or convert contaminants. In those situations, it is often possible to contain the contamination as a final solution or as an interim measure until appropriate technologies become available.
Soils. Radionuclides from historical weapons production and nuclear testing, as well as from industrial uses of radiation, appear to be a good match for developing containment technologies. For example, containment is a promising technology for the management of radioactively contaminated soils beneath the large high-level radioactive waste storage tanks at the U.S. Department of Energy Hanford site in Washington State. Removing radioactive contamination from soil is problematic from a worker-safety standpoint, and it may create further contamination of equipment, containers, and surrounding areas. Efforts to develop effective physical containment technologies for soil contaminants are continuous.
Groundwater. Groundwater is not generally suitable for absolute containment; however, between containment and conversion is a technology known as reactive barriers. Reactive barriers intercept contaminated groundwater plumes and are constructed of chemically reactive materials (e.g., iron) that bind or convert dissolved contaminants. Reactions between the contaminant and the iron either immobilize or degrade the contaminant by altering its chemical form (redox manipulation).
Passive Cleanup
Passive remediation technologies are increasingly common in some applications, and take advantage of naturally occurring chemical or biological processes that degrade contaminants to less toxic forms. The accepted term for this group of technologies is monitored natural attenuation (MNA), which is the result of regulatory recognition that natural biological processes are capable of degrading certain contaminants under specific conditions and that dispersion may aid in achieving objectives. MNA is employed for the cleanup of organic contaminants such as petroleum hydrocarbons in situations where the longer time frame associated with MNA does not increase the risks posed by the contamination. MNA recognizes that, while these processes are possible, they must be monitored to insure that the expected progression actually occurs. In the State of Florida, MNA is being used as an approved cleanup action for some former dry cleaning sites. At these sites, natural processes are being monitored as they degrade chlorinated solvents from the former dry cleaning operations.
MNA is one example of major innovation in this area. Environmental cleanup is a dynamic field. Advances in science and engineering fuel innovative approaches and technologies, and advanced technologies provide greater capabilities in meeting the ultimate goal of a safer and healthier environment.
SEE ALSO A BATEMENT ; B IOREMEDIATION ; D REDGING ; E CONOMICS ; I NCINERATION ; L AWS AND R EGULATIONS , I NTERNATIONAL ; L AWS AND R EGULATION , U NITED S TATES ; ; N ONAQUEOUS P HASE L IQUID (NAPL) S UPERFUND ; T IMES B EACH ; U NDERGROUND S TORAGE T ANK ; W ATER P OLLUTION .
Bibliography
Page, G.W. (1997). Contaminated Sites and Environmental Cleanup: International Approaches to Prevention, Remediation, and Reuse. San Diego, CA: Academic Press.
Tedder, D.W., and Pohland, F.G. (2000). Emerging Technologies in Hazardous Waste Management. New York: Kluwer Academic/Plenum.
Testa, S.M., and Winegardner, D.L. (2000). Restoration of Contaminated Aquifers: Petroleum Hydrocarbons and Organic Compounds, 2nd edition. Boca Raton, FL: Lewis.
U.S. Department of Energy. (1999). Groundwater and Soil Cleanup: Improving Management of Persistent Contaminants. Washington, DC: National Academy Press.
Internet Resource
U.S. Environmental Protection Agency Superfund Program Web site. Available from http://www.epa.gov/superfund .
J. Michael Kuperberg, Christopher M. Teaf, and Heather V. Ritchie
A microbe discovered in the mud in the bottom of the Hudson River may solve the problem of treating groundwater contaminated with the industrial solvent TCA (trichloroethane). The microbe, which lives without oxygen, converts TCA into chloroethane, a compound that can more easily be removed from groundwater. The Environmental Protection Agency lists TCA as a contaminant of concern at 696 of its 1,430 priority cleanup sites.
Comment about this article, ask questions, or add new information about this topic: